If you see something bad hurtling toward you, run in the exact opposite direction.
There’s nothing especially unreasonable about this – it’s the standard fight or flight response that human beings have learned over millennia of biological evolution.
But ‘fight or flight’ is not necessarily a useful paradigm for responding to the complex global emergencies of the 21stcentury – or many other issues and challenges of our lives today. We need to be able to transcend ingrained ‘hunter-gatherer’ ways of thinking to understand and respond to the converging systemic crises we face as a species.
The ‘fight or flight’ mode has shrunk our perceptions of the scope of possible choices ahead over the next decades. One reason for that, as I argued in my last post, is that we remain trapped within the prevailing ideological paradigm associated with modern industrial civilisation. This paradigm is a form of reductive-materialism that defines human nature, the natural world, and the relationship between them through the lens of homo economicus – a reduction of human nature to base imperatives oriented around endless consumption and production of materially-defined pursuits; pursuits which are premised on an understanding of nature as little more than a repository of material resources suitable only for human domination and material self-maximisation; in which both human and nature are projected as separate and competing, themselves comprised of separate and competing units.
Yet this ideology is bound up with a system that is hurtling toward self-destruction. As an empirical test of accuracy, it has utterly failed: it is not true because it clearly does not reflect the reality of human nature and the natural world.
It’s understandable, then, that in reacting to this ideology, many environmentalists have zeroed in on certain features of the current system – its predatory growth trajectory – and sought out alternatives that would seem to be diametrically opposed to those regressive features.
One result of this is a proliferation of narratives claiming that the clean energy transformation is little more than an extension of the same industrialised, endless growth ideological paradigm that led us to this global crisis in the first place. Instead of solving that crisis, they claim, it will only worsen it.
Within this worldview, replacing the existing fossil fuel energy infrastructure with a new one based on renewable energy technologies is a fantasy, and therefore the world is heading for an unavoidable contraction that will result in the demise of modern civilisation.
In this post, however, I will prove that while this analysis contains important insights, this conclusion is overly simplistic and scientifically flawed because it ignores some of the most robust empirical data. Far from being a sober, scientific perspective, this view is itself an ideological reaction that represents a ‘fight or flight’ response to the current crisis convergence. In fact, the proponents of this view are often as dogmatically committed to their views as those they criticise.
This doesn’t mean that the scenario of collapse and contraction should be dismissed as an impossibility – rather, a whole systems lens allows us to recognise that this is only one possible scenario among several, but it's not the only one - and it's not necessarily the most likely one either. Which scenario we move into is not a foregone conclusion. It depends on our choices over the next two decades – which is why I have described them as the most pivotal in human history.
We don’t have enough materials for the clean energy transformation. QED?
Perhaps one of the most widely-promulgated misconceptions is the idea that we definitively don’t have enough materials or minerals on the planet to support a comprehensive global transition to renewable energy technologies.
One of the most widely-circulated pieces of research on this is a 1,000 page technical report published by the Geological Survey of Finland (GTK) and authored by Simon Michaux, an associate professor of geometallurgy at the agency.
I reported on some of Michaux’s previous work for VICE, where I covered an earlier report he produced for the Geological Survey of Finland finding that the global oil industry was about to enter a spiral of crisis due to a combination of internal economic and energetic factors. In hindsight, much of what Michaux diagnosed in that report still stands up today as we move into a world of expensive energy amidst signs that the US shale boom is slowing down.
That’s why, when Michaux writes something, I pay attention. So when he wrote about the clean energy transformation – claiming that the world lacked sufficient material resources to sustain it – I read his report with keen interest. I certainly wasn’t the only one. Michaux has presented this analysis around the world at some of the highest levels, including the IMF, the UN Economic Commission for Europe, and the European Commission.
So I was disappointed to find that Michaux’s new report was replete with false assumptions, outmoded generalisations and incorrect data. But I wasn’t entirely surprised. Michaux’s expertise is in studying incumbent energy and extractive systems – not in the fundamentally different dynamics of the disruptive exponential technologies that characterise the clean energy transformation.
In the below analysis, I go through some of the most egregious errors and false assumptions in Michaux's report, offering my own insights as well as drawing on the work of other experts.
Myth 1: EROI for renewables is negative
In his report, Michaux provided figures to explain the mineral inputs required to replace the existing fossil fuel system equating to 2019 consumption figures with a completely renewable system. He concluded that to replace 46,423 power stations run by oil, coal, gas and nuclear power would require the construction of 586,000 power stations run by wind, solar and hydrogen.
Underlying these calculations is an overarching assumption that massively underestimates the Energy Return On Investment (EROI) of renewables compared to fossil fuels. Michaux claims for instance that:
“A consequence of the above is that renewable energy sources tend to have lower ERoEI ratios compared to fossil fuels, which means that these systems will have to work harder to replace fossil fuels. Thus, future non fossil fuel energy systems are likely to have a lower energy productivity compared to fossil fuel equivalents that they are replacing.
So, it follows then that in order to replace each fossil fuel power station, many more renewable systems will be required to be operating than current thinking assumes. This suggests that the future non-fossil fuel energy system may well be smaller in capacity than the current fossil fuel supported energy system.”
Michaux doesn’t provide a systematic analysis to justify this verdict on EROI, but one of the reviewers of his report acknowledged in the introduction is Pedro Pietro, a co-author of several EROI studies criticised for incorrectly underestimating the EROI of renewables. As Ron Swenson points out in a study published by Energies, Pietro’s low EROI calculations were based on flawed assumptions using “economic value as a proxy for energy”. Derived from the Spanish economy which is heavily dependent on imported fossil fuels, this resulted in an “inherently distorted” analysis “amplified by the ‘economic’ framework (i.e., energy policy) in which any given project is embedded”.
Lower EROI estimates of renewables based on this limited framework have been refuted by several meta-analyses in the scientific literature. According to one of the latest peer-reviewed meta-analyses led by Professor David J. Murphy, departmental chair of environmental studies at St. Lawrence University in New York and a pioneer of EROI who was taught by the concept’s founder, Professor Charles Hall, wind and solar actually have far higher EROI values than fossil fuels.
While the EROI values of wind and solar are “at or above 10”, the average EROI estimate for oil is about 4.2. Murphy et. al’s research concludes that many EROI analyses incorrectly compare fossil fuels with renewables by measuring them at the wrong areas. By consistently measuring them both at their ‘point of use’, they are able to develop a far more consistent approach.
These findings are corroborated by other researchers. Dr Paul Brockway at the School of Earth & Environment at the University of Leeds, whose team found in 2019 that EROI of fossil fuels was 6:1 and declining, with renewables therefore likely to be already higher.
While Michaux's actual calculations are not directly derived from EROI, his calculations showing an increased material intensity compared of renewables compared to fossil fuels would only be consistent with his negative EROI assumptions for renewables. However, those negative assumptions are contradicted by very good research, which suggests that his calculations cannot be correct. Higher EROI figures for renewables would suggest that less material inputs are required for the same energy output rather than more.
Myth 2: Coal is more efficient than renewables
Based on these false EROI assumptions, Michaux argues that “replacing fossil fuels will take more work than previously thought”. The implication is that, with EROI of renewables falling into the negative domain, there is a risk of actually using more energy to transition to renewables than renewables are able to generate.
So Michaux then attempts to give some examples showing how switching to renewable sources will be more energetically and materially intensive:
“For example, to replace a single coal-fired powered station of average size (average coal fired plant in 2018 was 861.3 MW installed capacity, producing 7.0 TWh of annually), 213 average sized solar PV array farms (33 MW installed capacity, producing 33 GW annually) would be required. Similarly, it can be calculated that it would take 87 wind turbine array farms of average size (37.2 MW installed capacity, producing 81.2 GW annually) to replace that fired power station”.
These figures are offered without any substantiation. Michaux uses them to suggest how materially intensive the transition will be – to replace just one coal fired plant we’d need to build 213 solar farms, or 87 wind farms. The subtext of course is that renewables are far less efficient and more expensive in real physical terms than fossil fuels.
Yet these figures are completely misleading and mean very little. The analysis is premised on the simplistic assumption that fossil fuels are more concentrated and renewables far more diffuse. Yet that assumption ignores the vast infrastructure and land footprint for mining and processing that is involved in the running of a coal plant. In the US, for instance, 8.4 million acres of land have been surface-mined for coal, a process requiring significant energy inputs. In other words, Michaux is committing a category error typical of many faulty EROI comparisons of fossil fuels and renewables that fail to examine their energy inputs and outputs in the same way.
In reality, calculations done a decade ago showed that a solar thermal plant can produce 18 Gigawatt per hour (GWh) per acre of land over a 60-year period; compared to a coal plant producing 15GWh per acre of mined land. The gap has widened since then because the efficiency of solar photovoltaic (PV) technology is improving exponentially, while coal is experiencing mounting costs and geological challenges.
Unlike fossil fuels, the costs of solar, wind and batteries have decreased exponentially at a rate of nearly 10% per year for the last few decades. Since the first commercial use of solar panels in 1958, their costs have dropped by a factor of 5,000. No wonder 99% of coal plants in the US are now more expensive to run compared to replacing them with wind and solar.
Another meta-analysis in Renewable and Sustainable Energy Reviews puts the EROI of solar photovoltaics at between 9 and 34:1, depending on the type of materials and technology deployed. As solar technology is continuously improving, the study concludes that “it is likely for PV technology to catch up to the maximum EROI from coal in the future.” Coal’s maximum EROI a century ago was about 80:1 – today, the EROI of oil, gas and coal is hovering between 6:1 to 3:1.
All this means that Michaux's positing of the EROI of renewables as less than fossil fuels, and in the negative, is wrong.
Myth 3: We have to replace the vast bulk of the primary energy supply
Michaux's report claims that the task ahead is to replace existing global primary energy supply from fossil fuels with renewables, as if this is a one-for-one substitution:
“In 2018, the global system was still 84.7% dependent on fossil fuels, where renewables (including solar, wind, geothermal and biofuels) accounted for 4.05% of global energy generation (Figure 12). At the very least, 84.7% of the primary energy supply will be required to be replaced with non-fossil fuel systems.”
Michaux is attempting to calculate a one-for-one substitution of the entire existing energy output of the current fossil fuel system. But this ignores the fact that a renewable energy system is an entirely new system with different dynamics, rules and properties.
Primary energy refers to the total energy embedded in fossil fuel production prior to its conversion by human activity into useful ‘final energy’. But the global economy doesn’t run on primary energy: it runs on the ‘final energy’ actually delivered to consumers to power our cars, keep the lights on and heat our homes. The difference between primary and final energy is in what the fossil fuel sector itself requires to keep itself going, along with huge transformation and distribution losses, all related specifically to the fossil fuel system.
To get from primary to final energy, a huge amount of energy is wasted due to those transmission and heat losses. In converting primary energy to electricity, nearly 70% of energy is lost to waste heat.
In contrast, solar and wind do not generate the same sorts of losses because they supply electricity immediately straight to the final energy stage. This means that Michaux’s calculations looking at how renewables should replace all of current primary energy are misguided, as we don't need to replace all of primary energy. So his estimate of the energy we need is already up to 70% too high. We don’t need to replace all of primary energy because solar and wind immediately produce final energy electricity, thus representing a different type of energy system altogether without the same type of energy waste.
The one-for-one nature of Michaux's calculations are clear from this paragraph of his report:
"In the year 2018, global oil consumption was 4662.1 mtoe (million tonnes of oil equivalent) (BP Statistical Review of the World Energy 2019). Converting mtoe units to TWh, global annual oil consumption in 2018 was 54 220.2 TWh (4 662.1 Mtoe = 54 220.2 TWh). Assuming that an ICE engine has an efficiency of 25%, this represents 13 555.1 TWh of useful work done. If this 13 555.1 TWh was supplied annually with just one of the replacements systems shown in Figure 6.34, how many average power plants would be required to be operational? Figure 6.7 shows the answer graphically."
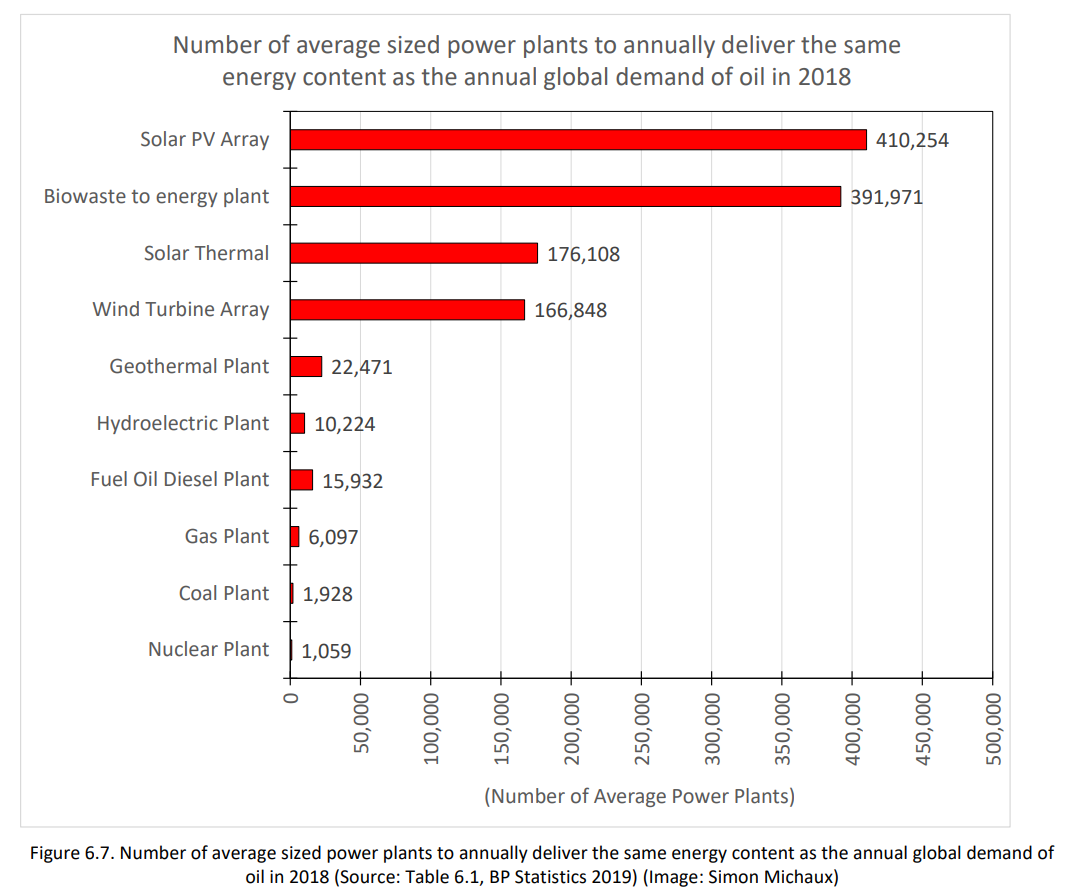
He then dedicates a whole section of the report to more sophisticated calculations around this, but the calculations are incorrect because the starting point is wrong. With up to 70% of primary energy lost in sustaining the energy waste heat before it reaches the useful final energy stage, that means that nearly 70% of the 4662.1 mtoe of global oil consumption was lost to waste heat in the process of converting the oil into useful final stage energy that we actually use for ICE's. If we rely on renewables, those losses don't occur, so we don't need to replace the equivalent, we need to replace some 70% less. The upshot is that in shifting to renewables, we will be able to do the same work, consuming up to 70% less energy than we are consuming today in the fossil fuel system, by eliminating those huge inefficiencies.
Myth 4: We need gargantuan quantities of lithium-ion batteries for stationary storage and electric vehicles
The main basis for Michaux’s findings that we will not have enough copper, cobalt, nickel, lithium and other metals for the clean energy transformation, is rooted in faulty assumptions about the sheer quantity of battery storage to phase-out fossil fuels. Several analysts looking at Michaux’s numbers have pointed out that they are totally wrong.
According to Matti Kehra, Senior Climate Specialist at the Finnish financial firm Nordea, Michaux’s assumptions about the scale of battery requirements in a new renewable energy system are grossly overinflated. This appears to create the massive demand for nickel and cobalt. Kehra points out that Michaux’s analysis is based on static battery chemistry assumptions from 2019. Yet changing battery chemistries and prices have already rapidly altered demand forecasts for such minerals. For instance, Bloomberg New Energy Finance’s (BNEF) 2019 forecast for cobalt demand was double the size of its 2022 forecast. Improved battery chemistries have meant reduced need for cobalt since then. This means that Michaux’s demand assumptions are already, at least, 50% too high.
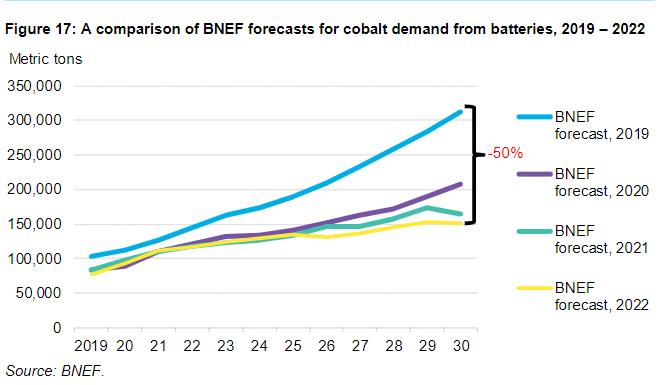
Vastly overestimating the scale of battery storage needed to accompany 100% global solar and wind generation underpins the foundations of much of Michaux’s overestimate of the materials and minerals inputs needed for the clean energy transition.
He claims that we need a 4-week stationary battery storage buffer alongside a 100% solar and wind system. However, according to Christian Breyer, Professor for Solar Economy at LUT University, Finland, a peer-reviewed hourly resolved global analysis in 145 regions suggests we only need 5 hours of battery demand on average. That's a huge difference, of course. Breyer comments: “The GTK approach seems to be not based on any credible state-of-the-art method.”
I will have more detailed comments on the storage issue below.
Michaux’s large overestimate is further justified by factoring in battery requirements for electric vehicles. But even taking that into account shows that his overestimate is ten times too high, as noted by Auke Hoekstra, who heads up Eindhoven University of Technology’s zero emissions research program.
Hoekstra also points out Michaux's incorrect assumption that stationary batteries use nickel, cobalt and manganese, “while experts will tell you they will use the heavier but cheaper and longer lasting LFP (lihtiumphosphate) batteries that use zero nickel and cobalt”.
So already battery chemistries are scaling which don't need nickel or cobalt, yet Michaux puts these minerals forward as an insurmountable bottleneck.
Michaux also ignores the potential to substitute copper with aluminium, which is 1,000 times more abundant than copper, and which is already increasingly being substituted in for use in power grids and car manufacturing over the last decade with further potential for much wider applications. Though by no means a perfect substitute, aluminium alloy is already being used for solar PV with great success. Further innovations that could increase aluminium's conductivity and make it cost-competitive with copper could be round the corner.
Since first publishing this piece, Auke Hoeskstra from the NEON research programme has put out an excellent analysis of how aluminium can now easily be substituted for copper without safety issues. Its slightly lower conductivity can be compensated for with 25% thicker wires which will still cost and weigh about half as much.
Myth 5: Lithium-ion is the only viable storage technology or battery chemistry
Michaux dismisses with no scientific basis the viability of other storage technologies which do not require lithium at all, and demonstrates a total lack of understanding of ongoing commercial innovation in the energy storage sector.
In one April 2023 interview about his report, Michaux claims entirely falsely that:
“Everyone knows what they want to do with a lithium battery, but no one knows what they want to do with a sodium battery.”
Yet that very month, the news had been announced that commercially viable sodium batteries for electric vehicles have gone into mass production in China. Months earlier, the first commercial sodium-ion batteries produced by another Chinese company were confirmed. Commercialisation of sodium-ion batteries has been continuing apace for some years. Commercial orders by industrial conglomerate ICM Australia of sodium batteries for stationary storage produced by Faradion took place as early as 2020. Michaux's dismissal of sodium batteries is nonsensical.
There are also other potential storage solutions not requiring lithium that Michaux wrongly dismisses. One of them is pumped hydro storage. According to Michaux:
“Due to the number of required power storage stations, it is impractical to plan for more pumped storage stations as they are very geographically limited.”
This is entirely false. Australian National University’s 100% Renewable Energy Group has published the Global Greenfield Pumped Hydro Energy Storage Atlas, which prove that the reality is the exact opposite of Michaux’s claim. The project identified 616,000 potential off-river pumped hydro sites (which can be easily designed to protect local ecosystems) around the world:
“... with storage potential of about 23 million Gigawatt-hours (GWh)… This is about one hundred times greater than required to support a 100% global renewable electricity system”.
The real global potential for pumped hydro storage is larger, as the analysis excludes existing reservoirs and old mining sites.
Due to its low cost, pumped hydro storage currently accounts for 97% of the world’s stationary electricity storage. As it is a mature, off-the-shelf technology, it can be easily be scaled up – eliminating any need for Michaux’s 4-weeks worth of lithium-ion battery storage.
When Michaux’s figures are re-evaluated removing this unnecessary scenario, by for instance assuming pumped hydro storage globally for stationary energy storage, his own figures for 2019 mineral production show that those production rates of key metals are more than sufficient.
This graph from Michaux shows his calculations of the total number of metals required to produce one generation of technologies to phase-out fossil fuels.
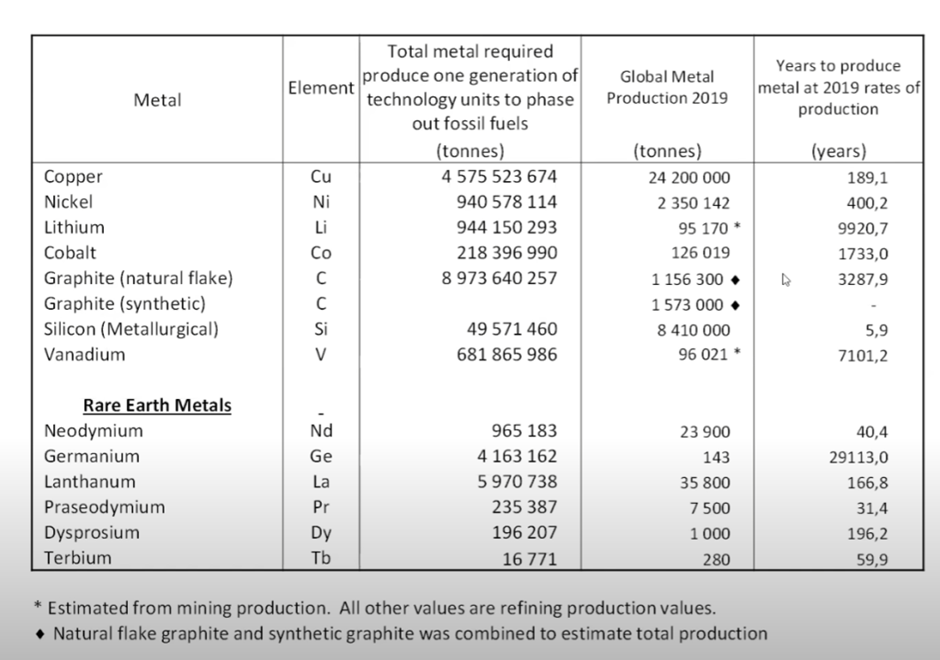
This graph provides Michaux’s breakdown of the metals by the different applications, such as electric vehicles and stationary battery storage.
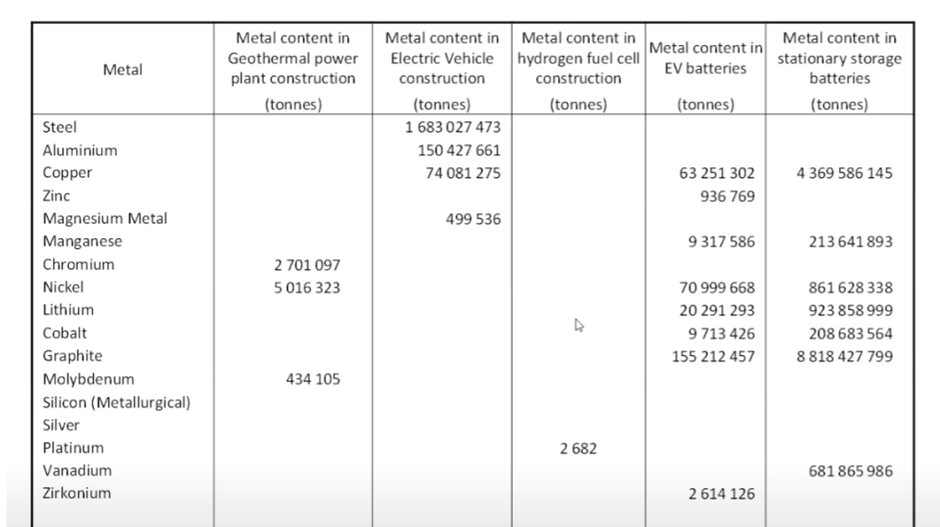
This provides the figures by which to calculate the total number of metals that might be needed according to Michaux’s highly overinflated estimates, when we remove the metals needed for 4-weeks of stationary battery storage. The following calculations were performed by the Australian ecological blog Eclipse Now:
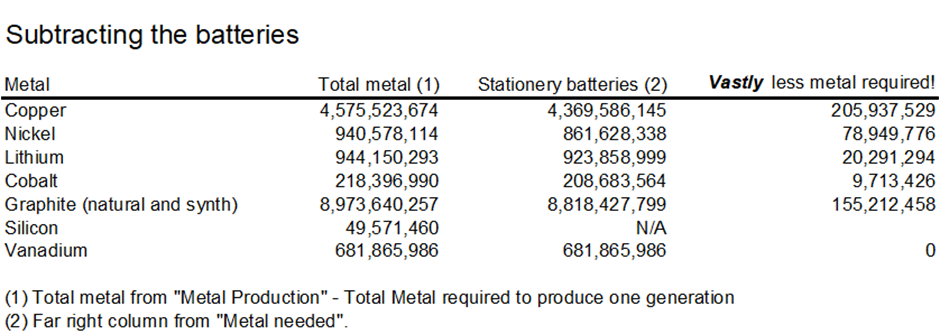
If we compare the figures on the right – the number of metals required by Michaux’s figures without the 4-weeks of lithium-ion stationary battery storage – with the figures for global metal production in 2019, there is more than enough copper, nickel and graphite. What about lithium and cobalt?
Michaux attempts to suggest limits to the production of metals by looking at 2019 production rates as if they will remain static. He uses this to claim it would take 213 years to mine the lithium we need for the clean energy transition. But that is not the case. For instance, his production rate for 2019 shows lithium at 95,170 metric tonnes. This is already outdated. In 2022, lithium production reached 130,000. This is a dramatic increase – in 2010 it was at about 28,100.
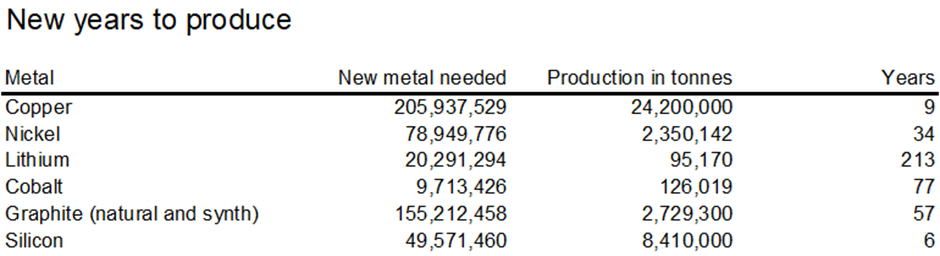
Total lithium reserves worldwide have been estimated by the US Geological Survey at about 26 million tonnes, with a total of 98 million tonnes identified as available lithium resources. Of course, if we just assume static 2019 production rates as Michaux does, it would seem reasonable to infer it will take 213 years to produce all the lithium needed. But that’s incorrect because as we’ve already seen, and as Michaux knows very well himself, metals production does not remain at one static rate but has been increasing exponentially over the last decades.
Since 2010, global lithium production has quadrupled over around a decade. Another more accurate way of looking at this is that it experiences a doubling every four years. At this doubling rate, we would close the gap on lithium requirements over the next three decades as the clean energy transformation accelerates.
Below is a simple projection of annual lithium production from 2022 to 2050 based on this doubling rate (an exponential pattern which we’ve seen throughout recent decades across different commodities including fossil fuels):
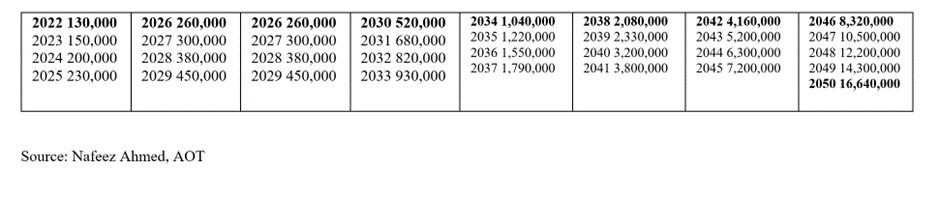
This simple projection suggests that by around 2042, cumulative lithium production would reach the (overinflated) 20,291,294 figure extrapolated from Michaux’s figures. In other words, even within Michaux's flawed assumptions, his own figures show that there are ample reserves for the renewable energy build out - assuming lithium-ion focused battery technologies for electric vehicles with pumped hydro storage for stationary battery storage.
In reality, many other options for both electric vehicles and stationary battery storage are already unfolding which do not require lithium and minimise nickel and cobalt inputs. Apart from sodium-ion batteries which are likely to become competitive with lithium over the next few years, and pumped hydro storage as already discussed, long duration iron flow batteries for stationary storage have reached commercial viability and are being deployed in Europe. The facts of technological development imply that in coming decades we will not be relying on just one single static battery technology, but most likely a mix of several deployed in different ways in different regions.
There are two further reasons to conclude that that there are more than enough metals and raw materials for the clean energy transformation: most conventional analysts have vastly overestimated the quantity of batteries that need to be deployed in the contexts of both a 100% solar and wind system, and the quantity of electric vehicles we need to manufacture.
Mistaken conventional assumption 1: Overestimating stationary battery storage by up to 90%
A number of scientific research groups including RethinkX where I was director of research communications have demonstrated with state-of-the-art models that the most optimal build out for a solar, wind and battery system in any region requires supersizing the generating capacity by about 3-5 times larger than demand.
Global energy firm Wartsila found that overbuilding solar and wind by four times peak load requires no seasonal storage, and needs only 4-10 days of multi-day storage capacity, making it the least cost system. Marc Perez at Columbia University found that building out overcapacity of solar and wind by a factor of three times bigger than demand not only dramatically reduces the need for battery storage, but lowers the cost of electricity by as much as 75%, while eliminating intermittency challenges. Perez’s team did another study focusing on the state of Minnesota in the US which showed that this approach would reduce the battery input for seasonal storage by as much as 90%. RethinkX’s modelling of California, Texas and New England also demonstrated this dramatic reduction in battery storage by supersizing solar and wind generation by three to five times demand. RethinkX has also done internal unpublished modelling covering every major region of the world confirming the generality of these findings. Such a system requires between 36 and 90 hours of storage a year, which eliminates demand gaps.
This means that the most optimally-designed clean energy system produces more than sufficient power even in the darkest days of winter at far cheaper cost, and reduces the need for battery storage by as much as 90%.
And this does not pose problems for materials supplies: Supersizing solar and wind based on existing technologies by 3 to 5 times will require greater inputs of abundant materials like silicon, aluminium, steel, iron and fibreglass, as well as copper (which without exorbitant demand from overinflated battery storage estimates will also be available, though aluminium already has the potential to be substituted in).
This means that conventional estimates of stationary battery storage requirements in a global clean energy system are about 90% too high, and therefore that associated conventional estimate of metals requirements to support that level of stationary battery storage are also too high. Michaux's analysis amplifies these already overinflated conventional assessments by several orders of magnitude.
In other words, Michaux’s overestimates of battery storage requirements go in completely the opposite direction of reality.
If we adopt the most optimal deployment strategy for solar, wind and batteries, we will not need the quantity of lithium and copper, for instance, that Michaux assumes. Further, if we were to use a careful mix of stationary storage technologies including lithium-ion, sodium-ion and pumped hydro, the quantity of metals needed for lithium batteries could be reduced dramatically depending on the deployment strategy selected.
Michaux also ignores the fact that as electric vehicles will be stationary 95% of the time, their batteries will be able to contribute to battery storage for the grid, which in some cases could entirely remove the need for additional stationary lithium battery storage.
For instance, one study of Australia which noted that pumped hydro could cheaply supply all of the country’s energy storage needs in a completely solar and wind powered system, also noted that if all Australia’s registered cars were replaced with electric vehicles, together they could supply some 900GWh of usable storage which is twice the storage needed in their pumped hydro model.
Mistaken conventional assumption 2: Overestimating electric vehicle battery storage by 80%
Michaux also wrongly assumes that it is necessary to replace “all fossil fuel-based vehicles with Electric Vehicle Technology (EVT)”, and therefore derives his figures for EV batteries from the need to replace the entire existing total fleet of 1,416,528,615 vehicles including cars, trucks, lorries and so on.
However, in 2017, Tony Seba and James Arbib of RethinkX released their groundbreaking analysis showing that the economic dynamics of the EV disruption would lead not only to the disruption of the internal combustion engine (ICE), but also to the disruption of private car ownership.
As with the disruptive technologies of solar, wind and batteries, electric vehicle costs are declining exponentially, and broadly on track to continue declining due to economic factors like technology learning curves and increasing production efficiencies. Projecting these cost reductions forward reveals that within the next two decades, owning and managing your own car would be more expensive than ordering an EV in a ‘Transport-as-a-Service’ model. That means the EV disruption will not only bring about the end of internal combustion engines, but incentivise the demise of private car ownership and the emergence of car-sharing and ride-sharing simply because it will be far cheaper.
Seba and Arbib further showed that these economic dynamics would lead to a mix and blurring of private and public transport models. Their modelling demonstrates that in a TaaS model, the number of cars required to service societal transport needs would be massively reduced because instead of everybody owning their own private car which for most hours of the days sits completely unused, it would be far cheaper for cars to be shared among citizens. In their model, they showed that the entire US vehicle fleet under TaaS would shrink by 80% - which means that the presumed metals requirements for the EV disruption will be 80% lower than conventional analysts presume.
This model, Seba and Arbib argued, would be accelerated by the advent of self-driving technology. Despite popular criticisms and legitimate caveats about self-driving disasters, autonomous vehicles are already being deployed in trial form for the public in several major cities. Two dozen states in the US already allow commercial deployment of intrastate autonomous trucks, which are now on the road in some areas, though usually drivers are still required for safety.
RethinkX predicted that self-driving cars would become ubiquitous within about a decade of regulatory approval, which was pinpointed to happen around 2020. While progress has been made, that time is coming later than their report initially anticipated.
A new report by the Victoria Transport Policy Institute (VTPI) concludes that that ‘Level 5’ autonomous vehicles “able to operate without a driver, may be commercially available and legal to use in some jurisdictions by the late 2020s, but will initially have high costs and limited performance”. They will become more “common and affordable” by the 2040s through to 2060s, with perhaps half of the vehicle fleet being autonomous by 2045. The report concludes that as safe and reliable Level 5 autonomy is likely to take a few more years to arrive, testing and regulatory approval may happen by around 2030, in which case by then “autonomous vehicles may be commercially available and allowed to operate in many areas”. RethinkX’s model shows that autonomous driving will become ubiquitous within a decade of regulatory approval. That suggests this could happen earlier than VTIP assumes, as early as in the period from 2030 to 2040.
In any case, between now and then, these studies reveal that we have a variety of potential pathways to accelerate the emergence of a clean electricity-based transport system. The dominance of a TaaS model involving a mix of shared and public transport options is something that we can choose to actively develop as societies, rather than simply waiting for passively. It represents a potential future of 80% less cars on the road, less congestion, no air pollution and increased cheap mobility available to all.
This means that Michaux’s assumption that we need to proceed with a one-for-one substitution of the entire incumbent vehicle fleet is simply wrong. We can instead design an entirely new and different clean transport system that will require 80% less metals inputs than conventionally assumed.
So in summary...
Michaux’s assumptions that we need 4 weeks worth of stationary battery storage are undermined by dozens of modelling studies based on empirical data from real-world solar and wind installations, which have shown that between 6-8 hours of storage is usually all that’s necessary to address intermittency challenges. Further research by RethinkX, Wartsila, Perez, and several other research groups suggests that supersizing wind and solar can reduce battery storage requirements further. All in all, the most robust figures suggest variations between about 30 to 90 hours of storage a year depending on the region. Michaux’s assumptions are further undermined by excellent data showing that pumped hydro storage alone can supply the world’s storage needs several times over, theoretically eliminating any need for stationary battery storage at all.
In such a scenario, I’ve shown that looking at Michaux’s own calculations suggests that there are more than enough copper, lithium, nickel cobalt and other metals supplies even if we were to replace the entire existing global transport fleet in a one-for-one substitution. In addition, the battery storage supplied by EVs will be able to play a major role in contributing to stationary storage requirements to balance the grid with solar and wind generation. Recall the Australian study showing how replacing the country's vehicle fleet with EVs would create such large storage potential it would be more double the capacity needed to cover the country's pumped hydro storage needs. Australia is very much a pathfinder case, and so we have good reasons to extrapolate from this to conclude that on a global scale, the quantity of lithium-ion batteries necessary to substitute the entire ICE fleet with EVs would be more than sufficient to supply storage needs for the grid. These sorts of counterintuitive dynamics and their implications are totally overlooked by Michaux.
However, even here, there won’t be a one-for-one substitution, as RethinkX’s research shows that the electric vehicle disruption will be able to eliminate 80% of privately owned cars which would reduce the metals requiring for the transport system substantially. In that case, of course, we would need more stationary storage solutions, but the good news from this analysis is that the evidence suggests even if we were to stick with lithium-ion, we will have more than enough overall.
In a LinkedIn response, Michaux has linked to this article in which he dismissed the studies showing that we don’t need anywhere near the scale of stationary battery storage that he claims. But he provides no serious reason for why his 4-week assumptions should hold, particularly as his own analysis makes no meaningful reference at all to the relevant solar and wind production data that those studies draw on. He also has no meaningful response to the conclusion above outlining a scenario involving largely pumped hydro stationary storage that totally eliminated his overinflated assumptions.
He also critiques RethinkX modelling showing the possibility of an 80% reduction in cars due to EVs and autonomous EVs, by arguing that since the new fleet of EVs will still have to travel the same number of miles and do the same work as before with a much tinier number of vehicles, this will only mean that the EV batteries will last for an equivalent far shorter period (80-90% less depending on how much the total number of cars drops). This means that the batteries will need to be replaced, by his calculations, in only a few years rather than 10-20 years.
If we take this argument as valid, it fails to add anything because once we eliminate his 4-week stationary lithium battery storage requirements, there is sufficient lithium by his own figures to replace and electrify the entire existing global transport fleet in any case which would potentially provide enough battery storage for the grid also. This means that the 80% reduction in cars we can aim for with EVs would simply be a bonus that massively alleviates lithium demand and any potential lithium bottlenecks, with it being possible to replace batteries much more quickly. However, we can also address this issue with far more efficient and stringent circular economy practices to recycle those lithium batteries, which would alleviate supply issues even further. As such a circular economy system will still take some years to develop, this shows that we will have sufficient time and materials over the next decade to establish that system.
And all of this is clear without even mentioning the fact that lithium batteries are likely to face competition from rapidly commercialising new battery technologies such as sodium-ion, iron flow, and beyond, within the next few years.
But there are also very good reasons to conclude that Michaux’s response to RethinkX’s transport scenarios is still trapped within the linear logic of one-for-one substitution thinking. That’s because when he calculates the global energy requirements to replace the entire global transport fleet, he fails to acknowledge that a new electrified transport system will have eliminated a huge quantity of the global shipping, logistics and transport infrastructure which is devoted precisely to transporting oil, gas, coal and iron ore for steel production.
The disruption and collapse of those industries will free up vast quantities of metals from the obsolete oil, gas, coal and ICE infrastructure including steel, aluminium, copper, nickel, cobalt and many other metals for recycling. This will also mean that at least around 40% of global shipping and logistics dedicated to transporting fossil fuels will evaporate. We can add to this iron ore for steel production because scrap steel and other metals will be cheap and ubiquitous; and as I’ve argued elsewhere, we can also consider the percentage of shipping devoted to livestock, grains, and so on related to conventional livestock agriculture which is also facing disruption over the next two decades from precision fermentation. Altogether, it’s plausible that some 70% of global transport for these commodities will disappear over the next two decades due to the energy, transport and food disruptions. And as Carbon Tracker have shown, the shift to metals and minerals extraction for electrification is nowhere the volume, weight and intensity as for coal, oil and gas (some 300 times less). This means that Michaux’s assumptions about replacing the entirety of the existing global transport miles to do exactly the same amount of work is false, as those miles are likely to drop as much as 70%.
This is a massive systemic transformation
Recognising the flaws in Michaux’s approach does not vindicate the idea that the current structures and value-systems of the global economy should simply stay the same. On the contrary, accelerating the energy and transport disruptions entails fundamental changes not only within these sectors, but in the way they are organised and managed in relation to wider society.
My critique of Michaux doesn’t justify complacency about metals and minerals requirements for the clean energy transformation. Resource bottlenecks can happen for a range of reasons as geopolitical crises like Russia's war in Ukraine make obvious. But there are no good reasons to believe that potential materials bottlenecks entail the total infeasibility of the transition. What it's important to do is to design and optimise the emerging system properly.
The key issue is that despite mountains of data, tables, charts, figures and numbers, what at first glance looks like a formidable and rigorous piece of research is based on questionable assumptions and poor methodologies. Michaux’s pessimistic verdict that the shift to renewable energy systems will be impossible to sustain because there are not enough metals and minerals is deeply flawed, and totally unravels because many of its most crucial assumptions are scientifically unsupported at worst or highly contested at best.
Michaux makes fundamental errors and adopts a range of completely unnecessary assumptions in his analysis of what a global phase-out of fossil fuels to renewables involves. He doesn’t appear to recognise that the new energy and transport systems are completely different systems with entirely new rules and properties, which will therefore have quite different dynamics – and that therefore this is not a one-for-one substitution of a bunch of products with another bunch of products in the same system, but a total shift in those systems.
Though widely publicised across social media and given an airing on many platforms of influence to policymakers, Michaux's research has also not been properly peer-reviewed. They are published as part of the Geological Survey of Finland’s 'working paper' series, which means that they are not scientifically verified and will not be used as the basis for policy decisions, but rather simply contribute to ongoing internal research.
Michaux has not retracted his analysis despite the variety of reasonable and scientific criticisms of his work. Indeed, in email correspondence with Michaux in October 2021, I wrote to him about many of the key disruptive system dynamics discussed here, including dramatically reduced metals requirements for dramatically reduced battery storage needs. He acknowledged that the approach I was using went against many of his fundamental assumptions:
“So I looked at Tony Seba’s work after reading your article, I found his ideas went against everything I had thought on several fronts. Then it occurred to me that the world he is describing is much nicer than the one conventional sustainability describes. I went away and had a good think about what was being proposed. So I have tried to incorporate his ideas in some of the scenarios.”
Although he admitted not necessarily agreeing with my approach, he was unable to offer any significant criticisms for why my alternative assumptions might be wrong. He wrote that he did not understand my approach to EROI (which is really just the approach advanced by David Murphy et. al), but acknowledged the plausibility of having much fewer cars, and stated that he would build a simulation model using different assumptions derived from RethinkX’s findings. He wrote:
"Anyway, I will build a simulation around what I call the Tony Seba set. Let’s see what happens. Raw material flows traced back against mineral resources. The outcome will most certainly be better than what we have now, but will it be good enough?... I still don’t understand your new approach to ERoEI. Then again this tool needs scrapping then reinventing into something practical."
Around a year later, Michaux described how his work had gone viral but explained his disappointment it was being used “inappropriately” by some pressure groups. He also admitted that he had not in fact tested his numbers against the models and research I had drawn to his attention:
"I have not had time to do any of the actions discussed below. I hope to get back to doing real work soon. Here is my latest presentation which seems to have gone viral if such a thing si [sic] possible."
Unfortunately, he did not use the opportunity provided by this correspondence to correct the mistaken direction of what he had already published. To the contrary, he has continued to repeat the same claims ad nauseum in interview after interview.
My critique of Michaux does not mean that concerns about metals and minerals inputs are irrelevant. It remains the case that there are entirely suboptimal design approaches to renewable energy and transport which could exacerbate the risk of bottlenecks and supply challenges. If we did indeed attempt to replace the entire existing vehicle fleet with electric vehicles, the numbers explored here show that we might well experience bottlenecks - although several experts have shown that Michaux's calculations on how we don't have enough lithium do not add up. If we restricted ourselves entirely to lithium-ion batteries and ignore the potential of pumped hydro for stationary storage, we would compound those risks. If we designed our solar and wind generating capacity incorrectly (with many focusing on trying to meet 80% of demand with the remaining met by other energy sources) we would create more expensive systems requiring much larger energy storage capacity.
In this sense, technology alone won't save us. We need to make good choices when it comes to which technologies we deploy, and how we deploy them - and how we distribute the benefits.
This also highlights the importance of a ‘circular economy’ approach to ensure maximum opportunities for recycling of materials. Lithium batteries for instance last up to 20 years, experiencing marginal decline after about 10 years of use. Recycling and repurposing old batteries will be essential; the same imperative of course will apply to solar and wind technologies (though perhaps less urgently as they now last for up to 50 years or longer).
We should also accelerate the build out of the new systems in partnership with people and communities. That means working with, not against, indigenous groups. It means doing so with respect for ecosystems, and ensuring that the extraction of resources is not done destructively, but regeneratively. Lithium mining for instance is currently notoriously unethical, environmentally destructive and exploitive. This is wholly unnecessary, and signifies that it’s not just about transforming our key carbon-polluting production systems, but also transforming our societal organising systems according to earth-centric values.
The opportunity to accelerate the ‘circular economy’ as an integral part of the emerging system will grow rapidly. As we increasingly disrupt incumbent fossil fuel systems, infrastructure, logistics, pipelines, oil rigs and beyond over the next two decades by accelerating this clean energy transformation, we will increasingly be able to access and recycle vast repositories of metals and minerals from these obsolete systems. Similarly, as we increasingly disrupt internal combustion engine vehicles over the next two decades, the vast quantities of scrap metal as old vehicle fleets become obsolete will open up the possibility of increasingly accessing and recycling vast repositories of metals and minerals from scrapped vehicles. These opportunities mean that there will be exponentially escalating scope to recycle materials from this old industrial infrastructure over the next two decades, which will provide further relief to materials pressures during the transition period. The good news is that in optimally-designed solar, wind and battery systems that generate three (and potentially up to ten) times the energy we generate today, we will have more than enough energy to support these emerging circular economy processes.
Getting a sense of perspective
I asked Dr Andre Manberger of the Faculty of Engineering at Lund University, Sweden, about Michaux’s work. Manberger was co-author of a major 2018 peer-reviewed study published in the Energy Policy journal which concluded that known mineral reserves “are sufficient to support the total level of solar power, wind power and electric motors”.
The paper noted that lithium may pose a limitation, but again it’s worth noting that the study pointed out that a variety of lithium-free storage approaches are likely to make this irrelevant. It's also worth recalling that this study adopted many conventional assumptions about the design of the new energy and transport systems which I’ve shown here may not hold.
Explaining his own research findings, Manberger told me:
“Overall, I think there is room for optimism when it comes to how rapidly many of the key transition technologies are developing. In many cases, markets respond to scarcity so that if a certain metal becomes more expensive substitutes are developed and material efficiency increase.”
Despite that, he concluded:
“I do agree with Simon that the current linear model is not sustainable in the long term. The earth is finite and we will have to use more recycled materials in the long-term. To make this possible may require actions now in the short term such as to design products that are more easy to recycle when they reach the end of their life and develop more circular business models”.
To an important degree, the different systemic dynamics of the new energy and transport systems will play a big role in incentivising and driving these very processes.
One near-term implication of the clean energy transformation is a massive reduction in logistics, shipping and materials throughput associated with the prevailing fossil fuel system – an issue I’ve discussed elsewhere. The new system will use 300 times less materials.
But Manberger also makes a crucial point. Even if we have sufficient materials to build out the first instantiation of a new global clean energy system, it will last for some decades before needing replacement. And that replacement process will require us to recycle and repurpose the materials we've already used.
Either way, we face the unprecedented opportunity and ecological necessity to move into a new system. This system includes the possibilities of abundant clean energy and transport with diminishing material throughput, requiring new circular economy approaches rooted in respect for life and the earth; and where the key technologies are so networked and decentralised that they work best with participatory models of distribution and sharing. This entails the emergence of a new economy with value measured in innovative ways, because traditional GDP metrics focusing on ever-increasing material throughput will become functionally useless.
This won't simply happen automatically. We need to make the right choices at every step of the way. Yet the system that all this entails will be a very different type of system to the one we inhabit today, and the terrain ahead to get there is going to be perilous. The claim that we cannot get to that destination because we don’t have the material resources to do so, however, is unfounded.
This article was amended on 6 May 2023 to add clarity and address claims in an article by Simon Michaux which purport to respond to certain criticisms of his calculations.
This article was amended again on 10 May 2023 to add further details on key issues. An article by Auke Hoekstra on aluminium substitution for copper was referred to, and further clarification on problems with Michaux's approach to EROI, primary energy, lithium-ion, and EVs were explored.